Like most deep-sea biologists, I have a large collection of decorated Styrofoam cups. A couple dozen line the bookshelf of my office, each displaying a rainbow of Sharpie colors. Each cup is painstakingly decorated with a cornucopia of deep-sea animals, equipment, and maps. Everything from giant squids to Alvin submersibles adorn the cups. I even have Cup-O-Noodles and New Orleans Daiquiri branded cups as part of the collection. Each cup is reminder of a deep-sea expedition and stands just 1-2 inches tall.
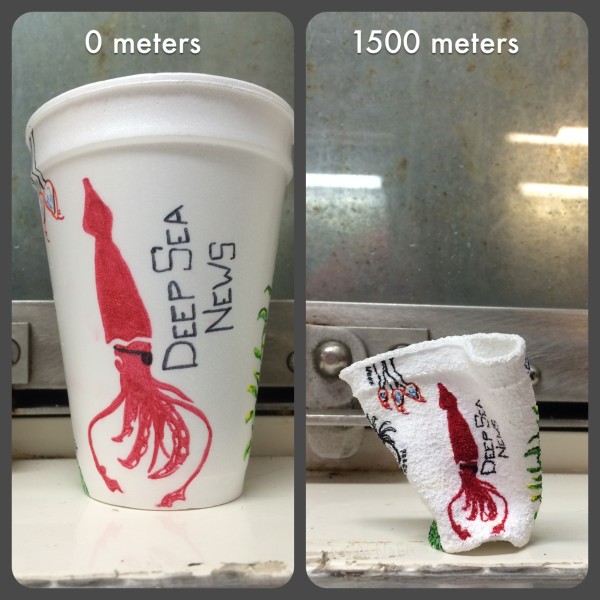
At the surface, each of those cups was 90% air and 10% tiny polystyrene beads. After each cup is artistically adorned with deep-sea imagery, they take a plunge to the abyss. At the crushing pressures of the depths, the air is squeezed out, leaving only those tiny polystyrene beads, and each cup becomes a miniature of its former self. One of the most extreme environmental gradients is the increase of pressure with increasing depth, starting at 1 atmosphere at the surface and reaching well over 1,000 atmospheres in the deepest parts of the ocean. How do organisms survive this great pressure, and what happens to them when they are brought to the surface?
The highest known pressure in the deep oceans coincides with the maximum known depth at the southern end of the Mariana Trench, the Challenger Deep. The actual maximum depth of Challenger Deep is disputed. The deepest reported measurement was by a Russian research vessel at 11,034 meters. The International Hydrographic Organization adopted 10,924 meters in 1993, but a subsequent 2010 survey, the most accurate to date, places the depth at 10,984 meters—25 meters greater. With every 10 meters corresponding to 1 atmosphere of pressure, this places the maximum known pressures in the deep between 1092.4 and 1103.4 atmospheres (110.7–111.8 MPa). Notably, several other deep-sea trenches—Tonga, Philippines, Kuril-Kamchatka, and Kermadec—in the Pacific Ocean also reach depths greater than 10,000 meters. In the Atlantic Ocean, the two deepest trenches are the Puerto Rico Trench at 8,800 meters and the South Sandwich Trench at 8,428 meters.
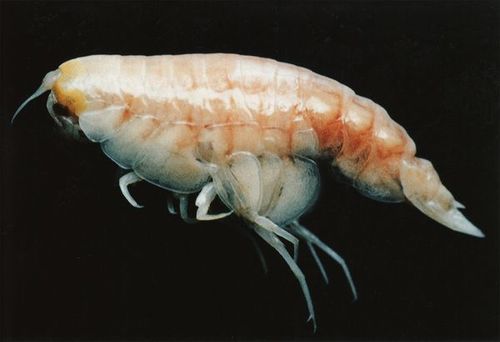
Exploring the depths of the ocean reveals a fascinating array of life forms, showcasing the incredible adaptability of both prokaryotic and eukaryotic organisms to high-pressure environments. Even in the Challenger Deep, where pressures exceed 110 MPa, microbial life thrives. Interestingly, several large multicellular organisms have been discovered at these extreme depths. A striking 74% of species found below 7,000 meters are endemic to these very deep areas. Among these invertebrates are the sea cucumber Myriotrochus bruuni, which inhabits the Mariana Trench at 10,710 meters, and the amphipod Hirondellea gigas, one of the most abundant creatures in the Challenger Deep at 10,897 meters. The hadal snailfish Pseudoliparis swirei holds the record for the deepest known vertebrate, living at an astonishing 7,966 meters.
How do organisms survive this depth? In short, there is a host of remarkable physiological and biochemical strategies that allow organisms to survive and thrive under the immense pressures found in the ocean’s depths. These adaptations include unique cellular structures and molecular mechanisms that confer resilience to high-pressure environments, such as specialized cell membranes, pressure-resistant enzymes, and unique protein folding techniques.
As you may remember from high school or college biology, a cellular membrane consists of a lipid bilayer. The structure is maintained entirely by the interaction of charges (or lack thereof) between water and the phospholipids. This makes the membrane semipermeable, much like a layer of oil on water. Extreme pressure results in tighter packing of the phospholipids, which lowers the permeability of the membrane. One adaptation by deep-sea animals to increase cellular permeability is to increase the percentage of unsaturated fatty acids. In a saturated fatty acid, all the carbons in the chain are linked by single covalent bonds. As you recall, a carbon can form four chemical bonds. If all these bonds are covalent (single), then a carbon could potentially attach to four other atoms. The term “saturated” comes from the fact that the carbon chain is loaded with hydrogens. If a carbon forms a double bond with another atom, it would bond with one less hydrogen. Thus, an unsaturated fatty acid has double bonds and is not “saturated” with hydrogens. The double covalent bond between adjacent carbons in an unsaturated fatty acid leads to a kink in the tails of the molecule. Thus increasing their concentration in the membrane leads to looser packing.
Pressure also selects for different enzymes. Changes in protein structure can influence their cellular function. Selection for rigidity is needed to counteract pressure and the resulting warping of proteins. Proteins contain hydrogen and disulfide bonds between different subunits and parts of the amino acid chain that dictate structure. Selection for proteins with increased bonding minimizes changes in shape due to pressure. Pressure can even make molecules more (or less) toxic. Urea is a good example: it becomes far more toxic as pressure increases. Deep-sea sharks, which like all sharks have a lot of urea in their blood, also have more of the protective chemical TMAO to offset this effect than their shallow-water cousins.
Pressure also does not favor air-filled volumes and sacs. The deepest diving submersibles require titanium spheres to hold the air-filled volume for their human inhabitants. For deep-sea animals, titanium is not an option, so most avoid having air-filled sacs. For example, deep-sea fish lack swim bladders. Other deep-diving animals like whales and seals have collapsible lungs to deal with extreme pressure (not to mention a whole host of other adaptations)!”